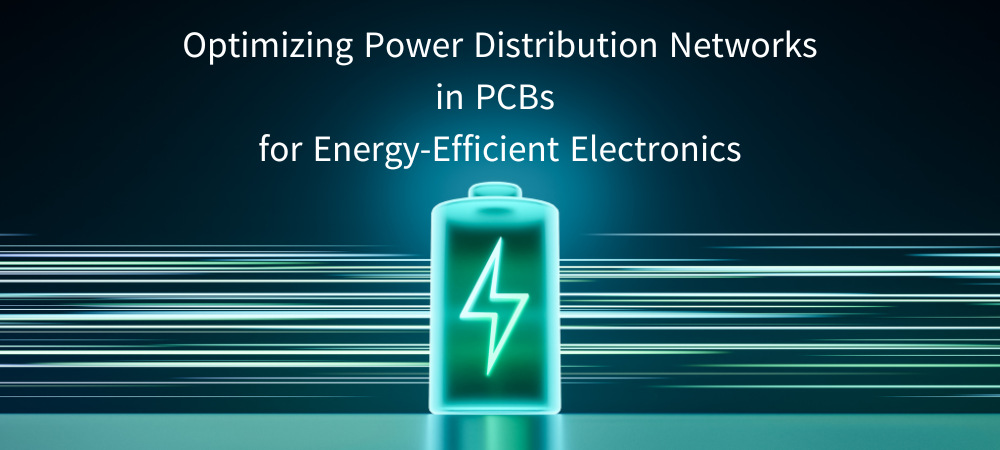
The downsizing revolution in electronics has resulted in significant advantages, including enhanced functionality, portability, and processing capacity. Nevertheless, the unwavering endeavor to create smaller and more efficient devices has imposed a substantial challenge on the design of Printed Circuit Boards (PCBs).
An essential element of this challenge involves optimizing the Power Distribution Network (PDN) to guarantee the effective transmission of electrical power across the board. Energy losses inside the power distribution network result in inefficient energy usage, reduced device performance, and challenges in managing heat. This article explores the complex strategy of optimizing Power Distribution Networks on Printed Circuit Boards (PCBs), with the ultimate goal of achieving energy-efficient electronics.
The role of Power Distribution Network in PCBs
Semiconductor devices necessitate sufficient power for proper operation. However, guaranteeing optimal power distribution is an intricate and expensive obstacle in the field of electronics design.
In order to maintain appropriate voltage levels for various components of the circuit, voltage regulators or converters might be strategically placed along this pathway. Within low-power designs, there may be switches present in the power supply that can be used to selectively deactivate specific areas of the circuit, hence lowering the amount of power lost due to leakage. In addition, capacitors can be incorporated to store sufficient charge to fulfill immediate demand during periods of high current demands, and then recharge during periods of lower current needs.
The PDN consists of a system of traces, planes, and components specifically intended to transmit power from the primary source to individual components. It has a crucial function in upholding the stability and dependability of electronic circuits by providing steady and pristine power. In the absence of a properly designed PDN, electronic equipment may encounter operational problems such as unpredictable behavior, data distortion, and even complete system breakdowns.
Designing an efficient and reliable PDN is paramount in PCB design. It involves meticulous planning and optimization to ensure proper power distribution and minimal interference. By focusing on PDN design, engineers can enhance the performance, longevity, and overall quality of electronic devices.
Overview of PDN parts and functions
The PDN comprises a critical subsystem within a Printed Circuit Board (PCB) design. It facilitates the efficient delivery of electrical power while mitigating unwanted noise. Here's a breakdown of the key PDN components and their respective functions:
- Power Sources: These represent the initial point of power injection into the PDN. They can encompass various configurations, including on-board DC-DC converters, external power supplies, or battery sources.
- Traces: Functioning as conductive pathways, traces route power from the source to individual components on the PCB. Their design prioritizes minimizing impedance and voltage drops to ensure efficient power delivery.
- Planes: PCB planes are extensive copper areas that serve as low-impedance paths for power distribution. They establish a stable ground reference plane and promote uniform power distribution across the board.
- Decoupling Capacitors: Strategically positioned throughout the PDN, decoupling capacitors function as local energy reservoirs and high-frequency noise absorbers. Their presence stabilizes voltage levels by mitigating voltage fluctuations arising from sudden current demands.
- Voltage Regulators: These integrated circuits (ICs) play a crucial role in regulating and controlling voltage levels delivered to sensitive components. They maintain voltage stability even with fluctuating input voltages or varying load conditions.
Figure 1: Ground plane in a PCB
Effects on PCB of poor PDN design
The Power Delivery Network is a critical subsystem for ensuring the stable delivery of electrical power throughout the PCB, thereby enabling the proper functioning of all electronic components. The PDN facilitates the distribution of power from the primary source, guaranteeing a consistent voltage supply to each component. Key elements constituting the PDN include conductive pathways (traces), interlayer connections (vias), extensive copper planes, and strategically placed decoupling capacitors.
These capacitors are meticulously positioned to minimize impedance within the network, ultimately delivering clean and stable power to sensitive components.
Figure 2: Decoupling capacitors (Source: Linkedin)
The ramifications of inadequate power distribution within a PCB design are far-reaching and can significantly impact circuit performance, reliability, and overall functionality. In the absence of a robust PDN, electronic components become susceptible to a multitude of issues, including:
- Voltage Droops: Insufficient power delivery can lead to transient voltage reductions during periods of high current demand. These voltage dips can induce malfunctions within components or even data corruption.
- Noise Interference: A poorly designed PDN may introduce unwanted electrical noise into the power supply. This noise can manifest as fluctuations in voltage levels, ultimately disrupting the functionality of sensitive components. Furthermore, the noise can propagate throughout the circuit, jeopardizing signal integrity and compromising overall performance.
- Signal Integrity Issues: Unstable power delivery can translate into signal integrity problems, such as ringing, overshooting, and undershooting. These phenomena can severely diminish the accuracy and reliability of data transmission within the circuit.
Figure 3: Example of signal ringing (Source: TI)
Guidelines for improving power distribution
Minimizing impedance
An essential principle in PDN design is to minimize the impedance between the power source and the active components on the PCB. High impedance results in a decrease in voltage that affects the devices, impeding their capacity to receive the required voltage for optimal functioning. The occurrence of this voltage drop might result in faults, decreased performance margins, and heightened vulnerability to noise.
Implementing a variety of methods is necessary to achieve low impedance. Firstly, employing wide trace widths with low resistivity materials such as copper enables enhanced current flow with little resistance. Furthermore, the inclusion of many power planes in the PCB design results in the formation of routes with reduced impedance.
These planes serve as integrated storage areas for electric charge, effectively minimizing the fluctuation in voltage that the components experience. The optimal number and configuration (solid, divided, or segmented) of power planes can be determined by considering the specific power delivery needs of the system.
Mitigating parasitics effects
Minimizing parasitic inductances and resistances is another important aspect of PDN improvement. These aspects, which are unintentional outcomes of the PCB layout, result in undesirable voltage dips and signal delays. High levels of inductance can result in sudden increases in voltage and oscillations during changes in current, which may result in harm to the device or unpredictable functioning. Similarly, a high level of resistance results in the dissipation of energy as heat.
Prudent trace routing solutions are crucial for decreasing inductance. Methods such as reducing the area of loops formed by the paths of electric current, using winding or zigzag patterns to increase the length of the path without sacrificing space, and placing decoupling capacitors near the power pins of delicate components all play a significant role in minimizing the inductive effects within the power distribution network (PDN). Similarly, employing low-resistance materials for traces and vias, together with assuring optimal via connections with high-quality plating, aids in reducing resistive losses.
Adding decoupling capacitors
Decoupling capacitors are essential for reducing voltage ripple and noise in the power distribution network (PDN). These capacitors are strategically positioned to serve as neighboring charge reservoirs, effectively diverting high-frequency fluctuations and maintaining a consistent voltage supply to adjacent components. The efficacy of decoupling capacitors is greatly influenced by their careful selection and strategic placement.
For optimal performance, decoupling capacitors should possess a low Equivalent Series Resistance (ESR) and a large capacitance value in order to efficiently reduce noise across a broad range of frequencies. By positioning these capacitors in close proximity to the power pins of the devices they are connected to, the inductive loop area is minimized and their ability to filter out unwanted noise is maximized. Utilizing numerous decoupling capacitors with varying capacitances in parallel is an advanced technology that can significantly improve the noise filtering abilities of the power distribution network (PDN).
Design tools
The pursuit of optimal Power Delivery Network design goes beyond conventional layout methodologies. Power integrity simulation programs have become an indispensable resource for engineers. These tools enable the modeling of the electrical characteristics of the Power Delivery Network, such as trace impedance, parasitic effects, and interactions with power planes.
Engineers can use simulations to analyze different design situations and detect any bottlenecks. They can also optimize trace width, plane arrangement, and decoupling capacitor placement before actually fabricating the system. By taking a proactive approach, the risk of design errors is minimized and the power delivery network (PDN) is guaranteed to meet the power delivery needs of the electronic device.
In the future, advanced technologies such as integrated power delivery modules (IPDMs) have the potential to greatly improve the performance of power delivery networks (PDNs). Integrated Power supply Modules (IPDMs) integrate power converter circuits with the Printed Circuit Board (PCB), reducing the negative effects caused by separate components and enhancing the efficiency of power supply. Furthermore, ongoing research is being conducted on new materials that possess even lower resistivity and enhanced thermal properties, which further advances the limits of PDN design.
Conclusion
Optimizing Power Distribution Networks (PDNs) on Printed Circuit Boards (PCBs) is a cooperative endeavor that necessitates meticulous evaluation of multiple elements. Engineers can assure effective power distribution throughout the PCB by limiting impedance, removing parasitic elements, intelligently deploying decoupling capacitors, and leveraging advanced design techniques. This results in greater device performance, decreased energy consumption, and improved thermal management. As electronic devices advance and require more power, optimizing power delivery networks (PDNs) will continue to be crucial for creating energy-efficient and dependable electronics.
Ultimately, the optimization of Power Distribution Networks (PDNs) on Printed Circuit Boards (PCBs) is a crucial element in the design of electronic devices that are energy-efficient. Engineers can achieve efficient power delivery throughout the PCB, resulting in improved device performance, reduced energy consumption, and enhanced thermal management, by minimizing impedance, reducing parasitic elements, strategically using decoupling capacitors, and employing advanced design techniques. As electronic devices continue to decrease in size and include more intricate features, the optimization of Power Distribution Networks (PDNs) will continue to be a crucial area of concentration in order to achieve electronics that are both energy-efficient and reliable.
22.08.2024
and get your PCBA quote within the next 10 minutes!